Exchange capacity
-
Exchange capacity:
-
Permanent charges
-
Variable charges
-
Al-oxides
-
Humus
-
Adsorption of cations and anions
-
Inner-sphere complex and outer-sphere complex
-
Cation exchange capacity (CEC)
-
Concepts
-
Methods
-
Comparison of CEC and ECEC
-
Organic soils
-
Variable-charge Ferralsols
-
Permanently charged Vertisols
-
Ambiguity: which method to use
1. Exchange capacity
1.1. Permanent charges
|
Fig.1 Isomorphic substitution |
(
Source: Brady and Weil 2002, p. 323.) |
-
The electric charge on clay particles results from isomorphic
substitution in the crystal lattices of clay minerals. One atom or element
fills a position usually filled by another of similar size without alteration
or modification of the lattice structure.
-
It takes place at the formation of minerals from lava flux
(not afterwards during weathering) due to deficiency or surplus of individual
elements in the lava flux.
-
The molecules fit by magnitude (size), but posses a different
charge.
-
However, as the number of O- or OH-ions in the tetrahedron or
octahedron is fixed, negative surplus charges are generated. Hence neutralizing
(counter-balancing) cations are inserted. They determine the cation-exchange
capacity
(
CEC)
and the nutrient
availability of soils.
-
Note: The charge deficit is permanent in the sense that it is
a structural feature of the clay particle and persists irrespective of changing
conditions such as soil pH and soil solution composition.
|
Fig.2 Clays |
(
Source: Brady and Weil 2002, p. 328.) |
Most of the permanent charge in soils resides:
-
on surfaces of layer-silicates mainly the 2:1 layer silicates
(illite, vermiculite, smectite)
-
the 2:2 clays (chlorite)
-
the 1:1 clays (kaolinite) -> contain only a small amount
of permanent charge on their planar surfaces
-
Note: surface varies from 10 m2/g
for Kaolinite to 800 m2/g for
montmorillonite.
|
Fig.3 Major Properties of Selected Soil
Colloids |
(
Source: Brady and Weil 2002.) |
1.2. Variable charges
-
Variable charges or pH-dependent charges depend on the pH of
the solution. They result mainly through protonation (release of protons) or
deprotonation of H+ from hydroxyl-groups (OH) at the
edges or surface of inorganic and organic colloids:
-
the edges of 1:1 layer silicate clays
(kaolinite)
-
on very poorly ordered aluminosilicates (allophane and
imogolite)
-
on crystalline hydroxides of Al (gibbsite) and Fe
(goethite, hematite)
-
OH combined with C of humus (SOM)
-
Thus, depending on the pH of the solution, the same location
produces both negative and positive net charges.
1.2.1. Al-oxides
-
Two examples of negative and positive charges of Al-colloids
are illustrated below.
|
Fig.4 Negative charges of Al-oxides |
(
Source: Brady and Weil 2002.) |
-
When pH raises, increase of hydroxylions (OH-) in the soil
solution. H+ ions dissociate and negative surface
charges result, leading to CEC (see fig.4).
|
Fig.5 Positive charges of Al-oxide |
(
Source: Brady and Weil 2002.) |
-
When pH declines, H+ ions in the
soil solution accumulate and protonation of H+ at
OH- ions take place. This results in positive
surface charges and thus the
AEC.
-
Note: Under normal to strongly acid conditions: Fe/Al-Oxides
and clay minerals have positive surface charges.
1.2.2. Humus
|
Fig.6 Humus |
(
Source: Brady and Weil 2002.) |
-
Humus has a small permanent negative charge and a large
pH-dependent charge.
-
Humus contains complex compositions with many active
groups.
-
The functional groups of humic materials contain carboxyl – and
phenolic groups which become dissociated at high pH.
-
A simplified schema depicts 3 main types with OH-groups which
are responsible for the high sorption capacity.
-
Similar to the sesquioxides and clay minerals negative and
positive charges develop based on loss of H+ of the
different groups.
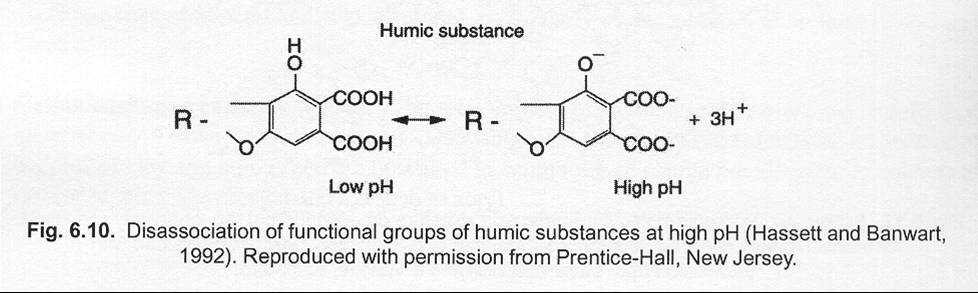 |
Fig.7 Dissociation of functional groups of humic substances
at high pH |
(
Source: Juma, 2002.) |
-
Example: dissociation of carboxyl-groups at high pH (see
fig.7).
-
Note: normally, the negative surface is higher than positive
one.
|
Fig.8 Humus components |
(
Source: Brady and Weil 2002.) |
|
Fig.9 Positive and negative charges of various soil
colloids |
(
Source: Brady and Weil 2002.) |
2. Adsorption of Anions and cations
|
Fig.10 CEC |
(
Source: Brady and Weil 2002.) |
-
Figure 10 shows how both anions and cations are adsorbed at the
same colloid.
-
Normally soil colloids have negative surface charges and are
enclosed by layer of cations.
-
Concentration is highest at the stern layer at the surface of
the clay minerals and lowest in the bulk solution.
-
Adsorption occurs via 2 possibilities: Inner-sphere-complex and
Outer-sphere-complex.
-
Inner-sphere-complex: For example
H2PO4-:
binds directly at negatively charged surface and cannot easily be
replaced.
-
Outer-sphere-complex: e.g.
Ca2+: water molecules build bridges between adsorbed
cations and negatively charged surface.
-
Results in weak binding through electrostatic attraction and
these ions can easily be exchanged or replaced.
-
Exchangeable cations are cations of the outer-sphere complexes.
They can be replaced by other cations.
-
This happens always charge-for-charge basis, e.g.
H+ and Na+
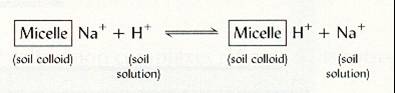 |
Fig.11 Exchange of Na |
(
Source: Brady and Weil 2002.) |
but 2 H+ and one
Ca2+.
 |
Fig.12 Exchange of Ca |
(
Source: Brady and Weil 2002.) |
-
This complex is named cation/anion exchange complex.
|
Fig.13 Ionic radius of exchangeable cations |
(
Source: Juma 2002, p. 194.) |
-
The adsorption is based on number of charges and the hydrated
radius.
-
The sequence of preferential adsorption is
Al3+ > Ca2+ >
Mg2+ > K+ =
NH4+ >
Na+.
-
Rule: the higher the charge and the smaller the hydrated radius
the stronger the attractive force between the cation and the surface (are less
easily removed).
Anion exchange capacity
|
Fig.14 Anion exchange capacity (AEC) |
(
Source: Juma, 2002.) |
-
Same principle as for
(
CEC)
.
-
Positive charges of Fe/Al-Oxides and SOM attract negative
ions like NO3- and sulfate
(SO43-).
-
Only variable-charge soils ever develop significant
AEC.
-
In tropics: When kaolinite and
Fe/Al dominate the
AEC can be higher than the
( CEC)
.
-
Important: impedes leaching of
NO3- from the
subsoil.
Methods
|
Fig.15 Determination of cation exchange capacity
(CEC) |
(
Source: Brady and Weil 2002.) |
-
(
CEC)
is an
important soil chemical property. It is used to: Classify soils and assess
their fertility.
-
The measurements of
(
CEC)
are rather empirical, and several different
analytical methods are used that yield different results. This depends
on:
1. The pH value at which the
( CEC)
determination is made. The
( CEC)
increases with increasing pH
(pH dependent variable charge).
2. The species of ions used in the exchanging or displacing
solutions, e.g. ion species differ in ease of displacement, and certain species
(K especially) may be trapped or fixed by some clay mineral species.
-
Standard method: In general, a concentrated solution of a
particular exchanger cation
(NH4+,
Ba2+) is used to leach the soil sample.
-
(
CEC)
can be
determined by:
1. Measuring the number of exchanger cations adsorbed (see
Fig.15 c +d).
2. The amounts of each of the elements originally held on the
exchange complex (Ca, Mg, Al, K, Na -> Fig.15 a+b).
3 types of CEC determinations are commonly
employed
1. NH4-saturation displacement method
(CEC7) conducted at pH 7.
2. Sum of cations method (CEC8.2) performed
at pH 8.2 in which the acid-generating H + Al (exchange acidity) are added to
the basic cations. This method is buffered against fluctuation and yields CEC
values higher than those from the NH4 saturation method
due to the fact that CEC values increase with higher pH in
OM, 1:1
clay minerals, and amorphous or short-range order minerals.
3. In the ECEC method the pH of the soil is not controlled and the
basic cations displaced by NH4 are added to the
KCl-extractable Al.
-
2 common methods are presented here: CEC buffered at pH 7 and
unbuffered method or effective cation exchange capacity (ECEC)
1. Buffered CEC:
-
Mostly with NH4 at pH 7 (sometimes with
Ba at pH 8.2).
-
If the native soil pH is less than the pH of the buffered
solution, the method measures not only the cation exchange sites active at the
pH of the particular soil but also any pH-dependent exchange sites that would
become negatively charged at pH 7.0.
-
This method measures potential or maximum CEC.
-
This method gives reliable estimates for temperate soils with
predominantly permanent-charge clays.
-
However, its reliability in many tropical soils is being
increasingly questioned as the artificially high pH of the exchange solutions
results in an overestimate of the variable-charge CEC.
2. Unbuffered method or effective
CEC:
-
The exchange takes place at the actual pH of the soil.
-
Thus, measures how many cations the particular soil can hold at
the natural soil pH.
-
The net negative charge of the exchange complex is neutralized
by exchangeable bases (Na, K, Ca, Mg) plus exchangeable acidity
(Al3+ , H+). The ECEC,
i.e. the sum of bases and exchangeable acidity is thought to represent the
soil’s cation exchange capacity at field conditions.
-
Important: Method used is be known. Both methods yield
significantly different values of CEC especially if the soil pH is much below
the buffer pH used.
Thumbnail Folie 34
Comparison of CEC and ECEC
|
Fig.16 CEC of various soil materials |
(
Source: Buol et al., 2003.) |
The following 3 examples explain the impact of the pH value at
which the CEC determination is made for
-
organic soils (histic epipedon),
-
variable-charged ferralsols and
-
permanently charged Vertisols.
a) Histic epipedon (according to Soil Taxonomy,
USA) has > 16 % OM
-
CEC of OM is very pH-dependent:
When the sample pH is at 3.7, the CEC increases greatly from
ECEC value to CEC7.
When the sample pH is at 6.4, there is no significant
difference between the ECEC value and CEC7
value.
b) Oxisol (Soil Taxonomy, USA) corresponds to
Ferralsol (WRB classification)
-
Predominant clay mineral in this soil is kaolinit. It is
greatly affected by the pH of the method (variable-charge soils).
At pH 5 + 5.7 = ECEC < CEC7 <
CEC8.2
c) Vertisol
-
Predominant clay in this soil is Montmorillonit. It is only
slightly affected by the pH of the method (permanent-charge soils).
ECEC = CEC7 =
CEC8.2
|
Fig.17 Charge Characteristics of Colloids |
(
Source: Brady and Weil 2002.) |
-
The example in Fig.17 explains how to calculate the
(
CEC)
of a soil when the amount of
the various colloids is known: 5 % SOM, 20 % Montmorillonite (Smectite) and pH
= 7.
-
Exchange capacity: = [SOM (%) x total charge] + [clay (%) x
total charge] = [(5/100) kg x 200 cmolc/kg] + [(20/100)
kg x 100 cmolc/kg] = 10 + 20 = 30
cmolc/kg
Ambiguity
-
Which calculation is correct or the right one? -Depends on
the purpose:
-
Potential CEC:
-
Used for soil
classification. Soil classification tries to utilize criteria that are
difficult to alter by common soil management practices such as lime
application.
-
Same conditions for all soils.
-
Shows degree of acidification
(e.g. CEC of 75 %: 25 % is occupied by H and Al)
-
ECEC:
-
More relevant for soil
fertility surveys and nutrient availability assays.
-
Indicates, what fraction
of exchangeable cations at the natural pH is occupied by basic cations.
-
Soil
fertility specialists prefer ECEC measurements because they reflect conditions
at the soil pH and can be manipulated by adding lime to the soil.
|